During the steelmaking process, while the molten steel is still in the ladle and before it is poured, the steel must be degassed in order to: (1) reduce/eliminate dissolved gases, especially hydrogen and nitrogen; (2) reduce dissolved carbon (to improve ductility); and (3) to promote preferential oxidation of dissolved carbon (over chromium) when refining stainless steel grades.
In the steel smelting process, unwanted gases are dissolved in the liquid, which could produce any number of imperfections and defects. A common method used to remove these undesired gases is vacuum degassing. The process is done after the molten steel has left the furnace and before being poured into ingots or introduced into a continuous caster (Fig. 1).
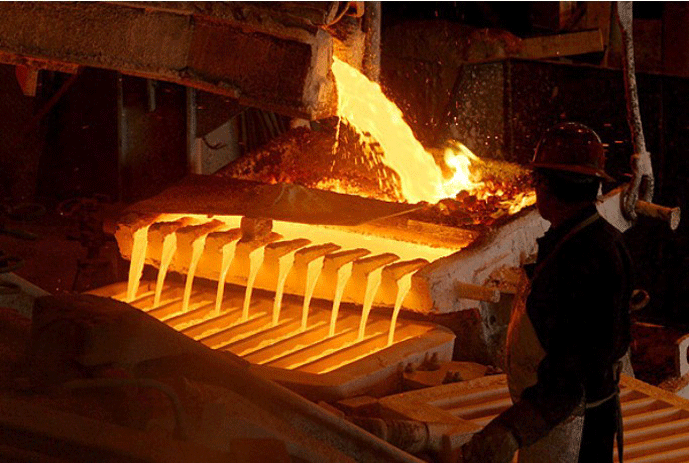
Vacuum Degassing Solves a Critical Problem
Throughout the early part of the 1900s, the steelmaking industry was plagued by poor quality steel This was due to the fact that during the production process, hydrogen and nitrogen gases were present in the molten steel. During cooling and subsequent solidification, the dissolved hydrogen caused pinholes and porosity in the final product since hydrogen has low solubility in steel at ambient temperature. The result was the release of hydrogen during solidification. Even the presence of a few parts per million of hydrogen gas causes defects and loss of yield strength.
Nitrogen also has low solubility in steel, under 10 ppm at ambient temperature. As the steel cools and solidifies, the nitrogen is rejected again resulting in defects in the final product such as embrittlement and reduced ductility. The solution was to pull a vacuum on the surface of the molten steel while it was heated, that is, to perform a vacuum degassing operation.
The idea of using a vacuum to remove dissolved gases from molten steel was initially proposed in 1940 by Soviet scientists A. M. Samarin and L.M. Novik. It was first tried industrially in 1952 at the Enakievskii metallurgical plant in the Soviet Union and in 1954 in Germany. By 1960, more efficient vacuum degassing methods such as the Dortmund Hoerder (DH) and Ruhrstahl-Heraeus (RH) methods became popular. They were followed in the mid-1960s by additional processes such as vacuum arc degassing, the ASEA-SKF process, and the vacuum oxygen decarburization process for high chromium steels. Soon after, this technology became an essential part of the steelmaking process and is now used throughout the world.
Vacuum degassing’s effectiveness in removing hydrogen and nitrogen from the steel is due to the reduced pressure between the molten steel and the vacuum, which causes the gases to become less soluble and separate from the liquefied steel. In the case of oxygen removal, the process is similar and is referred to as deoxidation, which is a chemical reaction between carbon and oxygen. The carbon combines with the oxygen, resulting in carbon monoxide (C + O = CO) gas. This carbon monoxide gas is then rejected from the surface of the steel in the same way as hydrogen and nitrogen. Since deoxidation, removes both oxygen and carbon molecules together, vacuum degassing is also an effective means to reduce the carbon content of the steel.
To better understand this phenomenon, it is useful to compare it to how water boils when placed under a vacuum. Bubbles form and float to the surface of the water, where they escape. In the same way, bubbles of hydrogen, nitrogen, and carbon monoxide form in the liquid steel under vacuum, rise to the surface and are removed by the vacuum system.
This technology allowed an economical way to commercially manufacture low carbon steel starting in the late 1960s.1 Beginning in the early 1980s vacuum degassing has seen increased use for the manufacture of ultra-low carbon steels with a carbon content of less than 30 ppm, as well as interstitial-free steels with carbon and nitrogen contents of 30 ppm or less. Further, deoxidation via vacuum degassing can provide low carbon steel with very low oxygen content. The resulting product has high ductility and is very homogenous. In addition to its metallurgical contribution, the vacuum degassing process improves the economics of high-quality steel production by allowing for a less expensive smelting process via a shortened smelting cycle as well as reduced consumption of alloying and deoxidant additives.
Vacuum degassing is a boundary layer phenomenon that occurs at the interface between the molten steel and the reduced-pressure atmosphere. Effective vacuum degassing depends upon sufficient exposed surface area of the molten steel. Vacuum degassing systems employ different methods to maximize this surface area. Two methods of vacuum degassing: ladle degassing and stream degassing are discussed here.
Ladle Degassing
During steel manufacturing, the molten steel is poured from the melting furnace into a vessel referred to as a teeming ladle (Fig. 2). In the ladle (aka tank) degassing method, the ladle is placed into a vacuum chamber, the chamber is sealed and a vacuum of 0.7 – 13.0 mbar (0.5 mm – 10.0 mm Hg) is applied. An alternate design uses the ladle itself as the vacuum chamber. In this design, the lid is designed with a vacuum tight seal between itself and the ladle. In order to ensure that the necessary surface area of molten steel is exposed to the vacuum, the steel is stirred so that new steel is constantly being brought to the surface. This is done by blowing an inert gas such as argon up through the molten steel (Fig. 3). The argon is introduced through the bottom of the ladle via a porous refractory plug. Additional stirring is generated by the deoxidation reaction, as carbon and oxygen are combined to form gaseous carbon monoxide, which forms bubbles that rise up through the molten steel and encourage mixing. There is a hopper with a vacuum lock on the cover of the ladle, referred to as an addition hopper. It allows for the addition of alloying elements during the degassing process. Desulphurization slag, commonly a mixture of CaO, MgO, MnO, and FeO, is also introduced through the hopper to remove any unwanted sulphur from the steel while under vacuum.
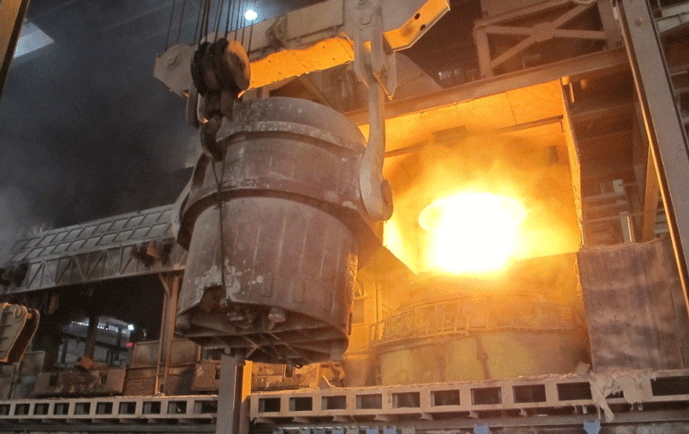
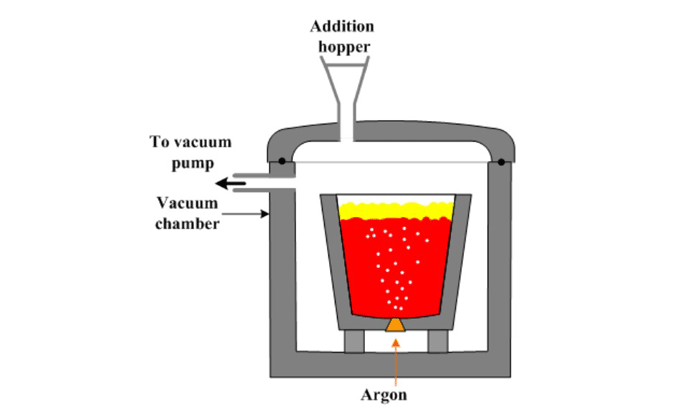
Stream Degassing
Stream degassing involves drawing a vacuum on the molten steel while it is being poured from a feeder tank (referred to as a tundish) to the casting mold. During pouring, the steel breaks up into droplets which greatly increases its surface area. Since degassing is so dependent on the exposed surface area, degassing happens very rapidly (Fig. 4) as the steel falls into the mold. The height the molten steel drops (i.e. the pouring height) is one of the most important variables impacting the effectiveness of the stream degassing process. In addition to ladle-to-mold degassing, there is another variation referred to as ladle-to ladle-degassing, in which a second ladle is located inside the vacuum chamber, in place of the mold. Due to the rapid nature of stream degassing, this method is used to pour ingots as large as 400 metric tons (880,000 lb.) or larger, requiring multiple ladle pours.
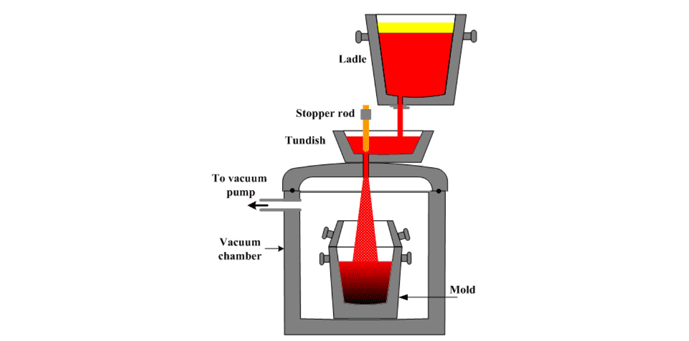
Vacuum Pump Selection
In order to select the best pump for vacuum gassing, several variables must be considered.
- The time available to draw the vacuum on the chamber, based on the production rate and other factors. A higher production rate requires a faster drawdown and higher capacity pump.
- The volume of dissolved gases to be removed from the steel. A higher degassing rate will require a more powerful pump system to maintain vacuum while pumping the liberated gases.
- Argon flow, in the case of ladle degassing. The vacuum pump has to remove not only the liberated gases but also the argon being used to stir the molten steel.
- The vacuum level required to provide satisfactory outgassing. This is affected by the steel chemistry and the gases being removed.
- The leakage rate of air into the vacuum chamber. Any air leaking into the system needs to be removed by the pump.
- The price of steam and electricity.
There are three types of pump systems used in vacuum degassing: (a) steam ejectors with necessary condensation stages; (b) steam ejectors in combination with water ring pumps; and (c) dry screw pumps, which don’t require a steam ejector.
Steam Ejectors
Steam jet ejectors use the venturi principle to create a vacuum by forcing steam through a nozzle. The nozzle provides controlled expansion of the steam, which effectively converts pressure into velocity, producing a vacuum. This draws in and entrains any gases present which enter at the bottom (Fig. 5). The steam is mixed with the pumped gases and passes out of the ejector.
Steam ejectors are commonly used in multiple stages for vacuum degassing by piping them in series, sometimes with condensing sections between them. While a single stage ejector can provide a 40 mbar vacuum, multiple stage ejectors can attain an ultimate pressure of 4 x 10-3 mbar. Steam ejectors have the advantage of no moving parts and correspondingly, high reliability and low maintenance. A major criterion when considering steam ejectors is the availability and cost of steam.
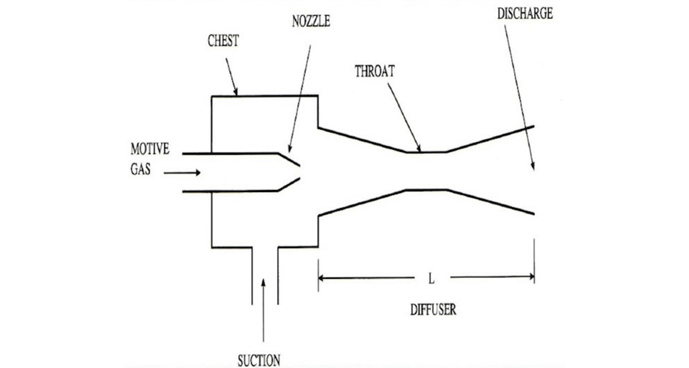
Water Ring Pumps
One of the vacuum pump systems used for vacuum degassing combines both steam ejectors with liquid ring pumps (Fig. 6) and involves putting several ejectors in series, followed by the liquid ring pump. The liquid ring pump is considered a positive displacement, wet style pump.
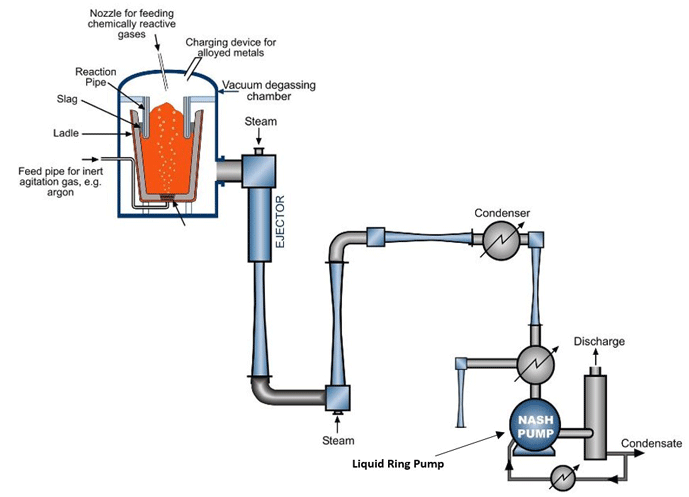
Like all wet style pumps, the liquid ring pump relies on a liquid to provide the seal between the rotating vanes and the pump housing. In vacuum degassing applications the pump is preceded by steam ejectors, which inject steam into the system. This steam condenses in the condensation stage producing a liquid, which is used as the pump seal.
The liquid ring pump is so named because of the ring shape the water takes due to centrifugal force (Fig. 7). The liquid ring results in a series of seals in the space between the impeller vanes, which form compression chambers. The eccentricity between the impeller’s axis of rotation and the pump housing results in a variation in the volume enclosed by the vanes and the ring. The result is that the gas is compressed and then discharges through a port at the end of the housing. These pumps can produce pressures as high as 30 mbar with lower pressures possible with liquids other than water. This type of pump has a pumping speed range of 25,000 to 30,000 cubic meters per hour.
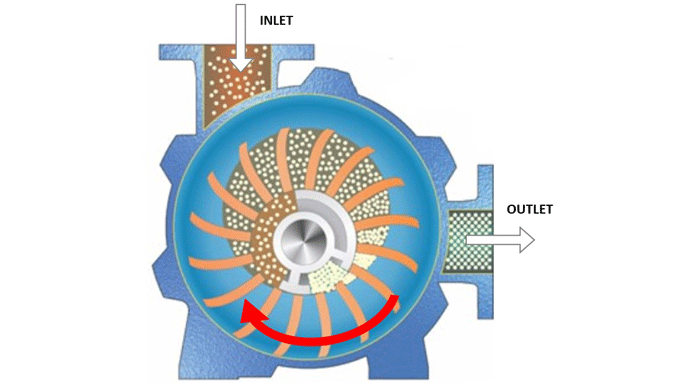
Dry Screw Pumps
Another pump system commonly used for vacuum degassing is the dry screw pump. The screw pump is a positive displacement, dry style pump. As a dry pump, it doesn’t require the introduction of a liquid into the system and therefore, is not preceded by steam ejectors.
The dry screw pump (Fig. 8) utilizes two rotating screws, one left-handed and one right-handed, that mesh without touching. The screws are machined to a tight tolerance and the space between them is very small. This tight tolerance, in combination with the motion of the rotating screw, impedes leakage of gas past the screws creating a seal. The rotation of the screws transfers the gas from one end of the pump to the other. The screws are designed so the space between them becomes reduced as the gas passes along, and it becomes compressed, causing a reduced pressure at the pump entrance. This pump features a high throughput capacity, good liquid handling, and tolerates dust and harsh environments. Typically, an ultimate pressure of 1 x 10-3 mbar can be achieved. Screw pumps have a pumping speed range of 50 to 539 cubic meters per hour.
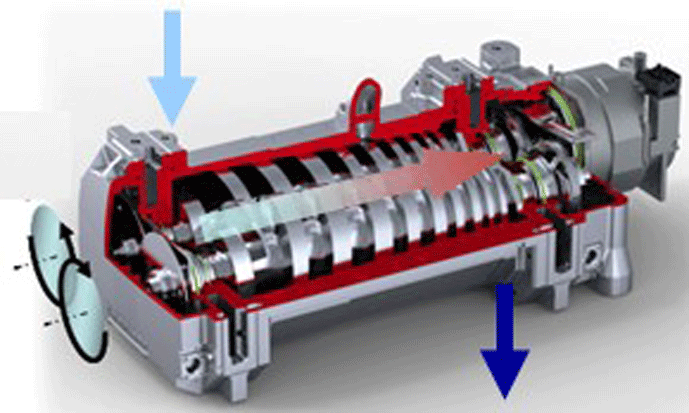
Summary
Vacuum degassing plays a critical role in the manufacture of steel. The vacuum degassing process allows the production of high quality, low carbon, and ultra-low carbon steels and stainless steels, with minimal oxygen, hydrogen, and nitrogen contamination. The main benefits include:
- Reducing hydrogen content to avoid embrittlement of steel.
- Reducing oxygen content to enhance microcleanliness.
- Improving the distribution of alloying elements and other additives.
- Controlling the composition of the steel to tighter chemistry specifications.
- Improving mechanical properties such as uniform transverse ductility, fatigue resistance, and high-temperature performance.
- Achieving exceptionally low carbon content steel heats beyond those obtainable by conventional means.
References
- Ispat Guru (www.ispatguru.com)
- NIPic (www.nipic.com)
- Nueva Mineria y Energia (www.nuevamineria.com)
- Substech – Substances & Technology (www.substech.com/)
- Gardner Nash (www.gdnash.com)
- Croll Reynolds (www.croll.com)
- Edwards Vacuum (www.edwardsvacuum.com