The role of materials science (Fig. 1) is to study, develop, design, and perform processes that transform raw materials into useful engineering products intended to improve the quality of our lives. It is said by many that material science is the foundation upon which today’s technology is based and that real-world applications would not be possible without the materials scientist. The discipline has expanded to encompass materials for many highly specialized product applications (Fig. 2).
.gif)
How Metallurgy and Materials Science Differ
The industrial revolution thrust metals into the forefront of technology, and they have stayed there ever since becoming the very foundation on which our modern society is built. One cannot envision a life where our transportation and communications systems, buildings and infrastructure, industrial machines and tools, and safety/convenience devices that are not an integral part of our daily lives.
Metallurgy is that part of materials science and materials engineering which studies the physical and chemical behavior of metallic elements, intermetallic compounds and their alloys. This definition is all-encompassing and includes the study of processes run in furnaces and ovens, the forging and rolling of metals, foundry operations, electrolytic refining, creation and use of metal powders, welding, heat treatment and much more. One might say that metallurgy is the application of knowledge of materials while materials science focuses on the theory of the structure, properties, processing, and performance of engineering materials.
.gif)
Metallurgy is also the technology of metals: the way in which science is applied to the production of metals (including heat treatment), and the engineering of metal components for use in consumer products and manufactured goods. The production of component parts made from metals is traditionally divided into several major categories:
- Mineral processing, which involves gathering mineral products from the Earth’s crust.
- Extractive metallurgy, which is the study and application of the processes used in the separation and concentration of raw materials. Techniques include chemical processing to convert minerals from inorganic compounds to useful metals and other materials.
- Physical metallurgy, which links the structure of materials (primarily metals) with their properties. Concepts such as alloy design and microstructural engineering help link processing and thermodynamics to the structure and properties of metals. Through these efforts, goods and services are produced.
What is Metallurgical Engineering?
Metals and mineral products surround us everywhere – at home, on our way to and from work and in our offices or factories. They form the backbone of modern aircraft, automobiles, trains, ships, and endless recreational vehicles; buildings; implantable devices; cutlery, cookware; coins and jewelry; firearms and musical instruments – the uses are endless. While threats abound from alternative material choices, metals continue to be at the forefront and the only choice for many industrial applications.
Today’s metallurgist works to develop new materials, new processes to make them, and test new theories and models to understand them. We have the means to measure properties at the macro, micro, Nano and atomic scales giving us unprecedented access to fuel new developments. The strong dependence of our society on metals gives the profession of metallurgical engineering its sustained importance in the modern world.
Classifications of Materials
There are literally thousands of materials available for use in engineering applications. Most fall into one of four broad classifications – metallic, ceramic, polymeric and composite (Table 1). For example, a subcategory of metallic ferrous materials is steels, which are typically divided into five groups familiar to most heat treaters, namely:
- Carbon steels
- Alloy steels (sometimes referred to as low-alloy steels)
- Stainless steels
- Tool Steels
- Special-purpose steels
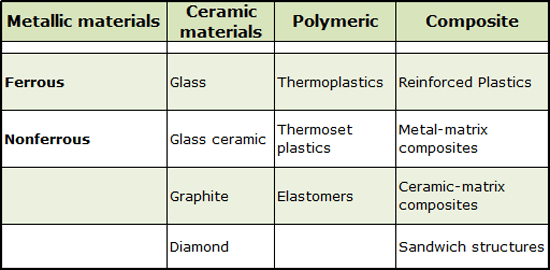
Today, many of these highly specialized materials have emerged as complements for or threats to metal’s dominance. Composites in the aerospace industry are one such example.
The Role of the Vacuum Heat Treater
Vacuum processing (Fig. 3) is one of the more specialized disciples that make up the field of heat-treating (aka heat treatment). What makes it unique is that the process is performed in a highly controlled environment (whether it be vacuum or partial pressure of a particular gas or gas mixture). In vacuum heat treatment the chamber in which the parts are placed is first evacuated of air before the onset of processing. Component parts are then processed under highly controlled conditions to a predetermined process (i.e. time-temperature-vacuum level) in order to create changes in the material’s properties (physical, mechanical, metallurgical).
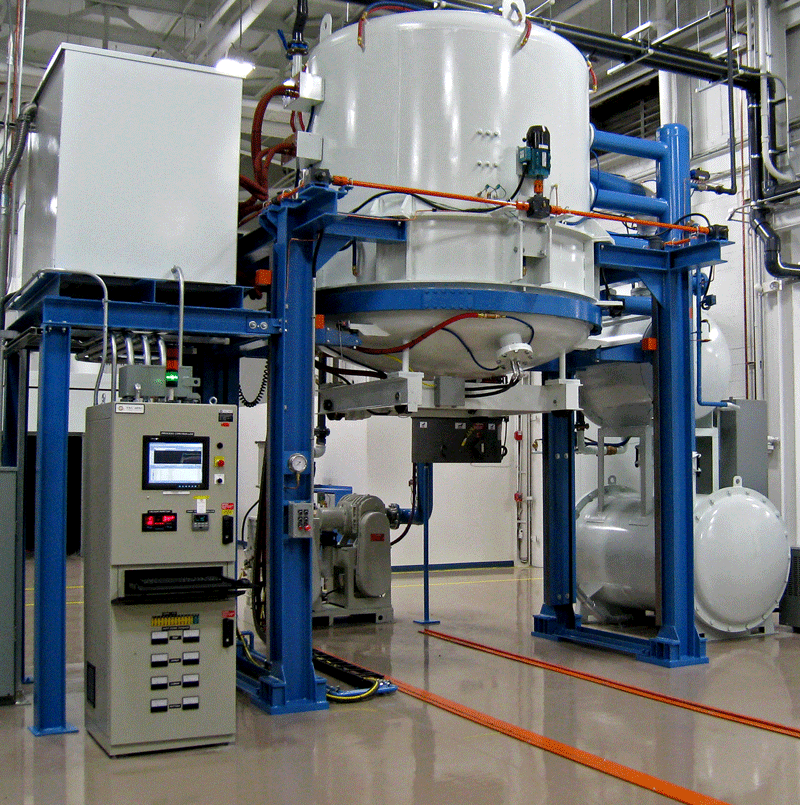
The vacuum heat treater is the individual responsible for operating vacuum equipment and observing the process being run so as to ensure that the heat treatment recipe was run correctly. Design, manufacturing, and quality engineers are responsible for oversight of the process to ensure that the component performance metrics have been achieved.
While a complete understanding of all metallurgical subjects is important, the vacuum heat treater needs to have a keen awareness of the incoming raw material characteristics (e.g., microstructural features such as banding, grain size, alloy carbides and the presence of surface decarburization) and the effects of the heat treatment process parameters on the final hardness and microstructure (e.g., martensite formation, non-martensitic transformation products, retained austenite, intergranular oxidation/intergranular attack, and mechanical properties such as toughness).
A wide variety of heat treatment processes are performed in vacuum such as; age hardening; annealing; brazing; case hardening; coating; hardening; metalizing; normalizing; solution heat treatment; stress relief and tempering to name a few. These are all designed to achieve a specific end result (e.g., higher strength, improved wear resistance, enhanced corrosion behavior). Many of these processes require temperatures in excess of 1000ºC (1832ºF) as well as specially designed vacuum furnaces and controls in which to perform them.
Vacuum processing and technology is very important in metallurgy; it prevents metals from oxidation when subjected to high-temperature treatments. Vacuum heat treatment is performed on both raw material and semi-finished goods such as tools, transmission gears, engine valves, and fuel injection systems.
Emerging Areas of Materials Development2
Vacuum processing is well positioned to meet the heat treatment needs of new “smart” (aka intelligent, state-of-the-art) materials, which will have a significant influence on many of our current technologies. These materials are designed to sense changes in their environments and then respond to these changes in predetermined manners, traits that are also found in living organisms. This concept is being extended to rather sophisticated systems that consist of both traditional and advanced materials. For example, actuators may be called upon to change shape, position, natural frequency, or mechanical characteristics in response to changes in temperature, electric fields, and/or magnetic fields. Shape memory alloys, piezoelectric ceramics, magnetostrictive materials, and electrorheological/magnetorheological fluids are being used for actuators.
In another example, one type of smart system is employed in helicopters to reduce aerodynamic cockpit noise that is created by the rotating rotor blades. Piezoelectric sensors inserted into the blades monitor blade stresses and deformations; feedback signals from these sensors are fed into a computer-controlled adaptive device, which generates noise-canceling sound.
Finally, until very recent times the general procedure utilized by scientists to understand materials was by studying large and/or complex structures and then investigating the fundamental building blocks of these structures. This approach is often referred to as “top-down” science. However, with the advent of scanning probe microscopes, which permit observation of individual atoms and molecules, it has become possible to manipulate and move atoms and molecules to form new structures and, thus, design new materials that are built from simple atomic-level constituents, that is to create materials by design. This ability to carefully arrange atoms provides opportunities to develop mechanical, electrical, magnetic, and other properties that are not otherwise possible. This “bottom-up” methodology has resulted in the creation of nanotechnology and we will see ever more increasing adaptations of this technology in the future.
Summary
It is believed by most, that our economic and technical progress into the 21st century will depend in large part on further advances in metal and mineral technology. For example, advancements in energy technologies such as the widespread use of nuclear fusion will only be possible by material developments not yet in existence. The future is indeed bright for today’s material scientists and those engineers who chose metallurgy as their career choices.
References
- Herring, Daniel H., Vacuum Heat Treatment, Volume I, BNP Media, 2012.
- Mobasher, M.D. and R. Azmi, Material Science & Engineering (mseru.blogspot.com)